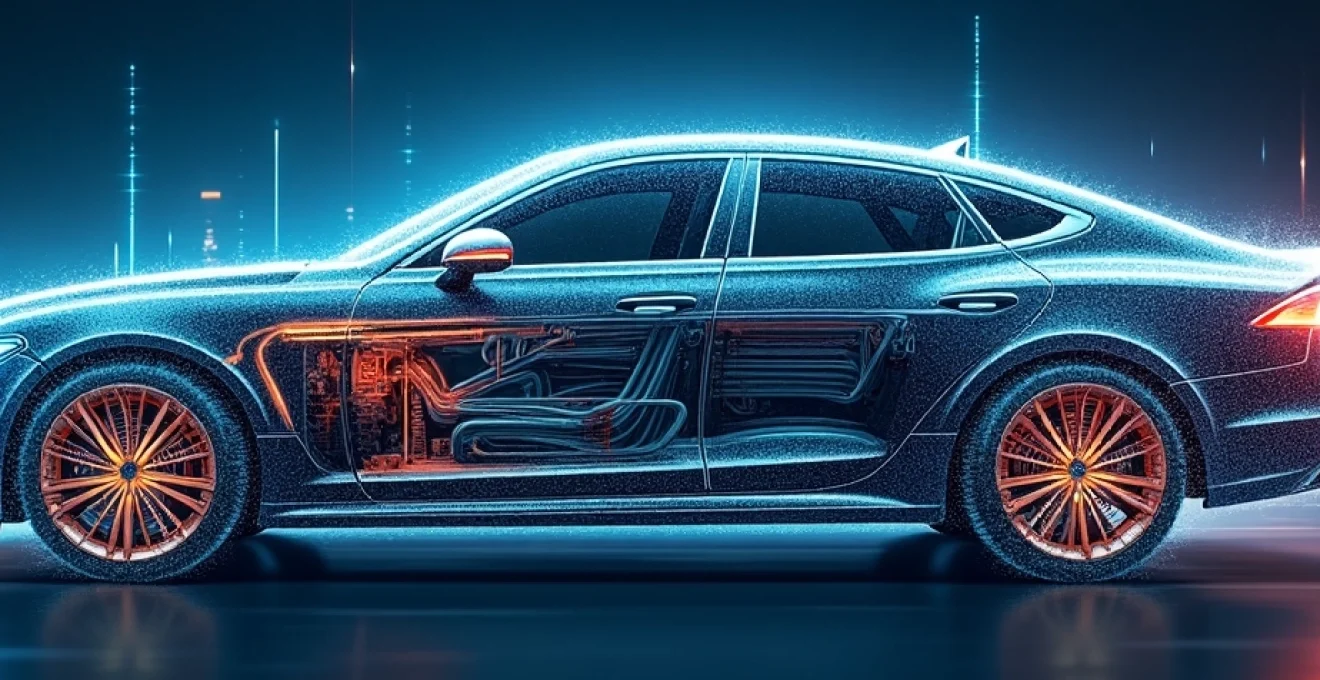
As fuel prices continue to fluctuate and environmental concerns grow, gasoline-electric hybrid vehicles have emerged as a smart choice for drivers seeking to maximize fuel efficiency without sacrificing performance. These innovative machines combine the best of both worlds, leveraging the power of traditional combustion engines alongside cutting-edge electric motor technology. By understanding the intricacies of hybrid systems, drivers can make informed decisions and truly harness the potential of these fuel-sipping vehicles.
Fundamentals of gasoline-electric hybrid technology
At its core, a gasoline-electric hybrid vehicle utilizes two distinct power sources: a conventional internal combustion engine and one or more electric motors. This dual-power system allows the vehicle to optimize energy usage, significantly reducing fuel consumption and emissions compared to traditional gas-only vehicles. The electric motor can power the car at low speeds or assist the gasoline engine during acceleration, while the combustion engine takes over at higher speeds or when additional power is needed.
One of the key components in hybrid technology is the battery pack, typically a high-voltage nickel-metal hydride (NiMH) or lithium-ion (Li-ion) unit. This battery stores electrical energy generated through various means, including regenerative braking and the gasoline engine itself. The sophisticated power management system constantly monitors driving conditions and energy demands, seamlessly switching between power sources or combining them for optimal efficiency.
The synergy between these components results in impressive fuel economy figures, with many hybrid models achieving over 50 miles per gallon in city driving conditions. This efficiency is particularly notable in stop-and-go traffic, where traditional vehicles waste significant energy during idling and frequent stops.
Advanced powertrain configurations in modern hybrids
As hybrid technology has evolved, manufacturers have developed various powertrain configurations to suit different driving needs and vehicle types. Each configuration offers unique advantages in terms of performance, efficiency, and cost-effectiveness. Understanding these different architectures can help you choose the hybrid system that best aligns with your driving habits and preferences.
Series hybrid systems
In a series hybrid system, the gasoline engine does not directly drive the wheels. Instead, it acts primarily as a generator, producing electricity to power the electric motor or charge the battery. The Toyota Prius Prime, a plug-in hybrid version of the iconic Prius, utilizes a variation of this system. When operating in EV mode, the Prius Prime can travel up to 25 miles on electric power alone, making it ideal for short commutes or urban driving.
The series configuration allows for a simpler drivetrain design and can provide smooth, electric-like acceleration. However, it may be less efficient at highway speeds compared to other hybrid configurations.
Parallel hybrid architecture
Parallel hybrid systems allow both the gasoline engine and electric motor to directly power the wheels. The Honda Insight employs this configuration, using its Integrated Motor Assist (IMA) technology. In the Insight, the electric motor is sandwiched between the engine and transmission, providing additional power during acceleration and recapturing energy during deceleration.
This architecture offers a good balance between efficiency and performance, making it well-suited for a wide range of driving conditions. The parallel system can be more efficient at highway speeds compared to series hybrids, as the gasoline engine can directly drive the wheels without the energy losses associated with converting mechanical power to electrical and back to mechanical.
Power-split hybrids
Power-split hybrids, also known as series-parallel hybrids, combine elements of both series and parallel systems. The Ford Fusion Hybrid utilizes a power-split configuration through its eCVT (electronically controlled continuously variable transmission) system. This setup allows the vehicle to operate in series mode at low speeds, parallel mode at higher speeds, and a combination of both when needed.
The flexibility of power-split systems enables them to optimize efficiency across a wide range of driving conditions. However, the complexity of these systems can lead to higher manufacturing costs and potentially more complicated maintenance requirements.
Plug-in hybrid electric vehicles (PHEVs)
Plug-in hybrid electric vehicles (PHEVs) take hybrid technology a step further by incorporating larger battery packs that can be charged from external power sources. The Chevrolet Volt, a pioneering PHEV, uses a series-parallel hybrid system with a substantial battery capacity. This allows the Volt to operate as a pure electric vehicle for up to 53 miles before switching to hybrid mode.
PHEVs offer the benefits of both electric and hybrid vehicles, providing zero-emission driving for short trips and the flexibility of a hybrid system for longer journeys. However, they typically come with a higher price tag due to the larger battery pack and more complex powertrain.
Regenerative braking and energy recovery systems
One of the most innovative features of hybrid vehicles is their ability to recapture energy that would otherwise be lost during braking or deceleration. This process, known as regenerative braking, significantly contributes to the overall efficiency of hybrid systems. By understanding how these energy recovery mechanisms work, drivers can maximize their vehicle's fuel-saving potential.
Kinetic energy conversion in Toyota's Hybrid Synergy Drive
Toyota's Hybrid Synergy Drive, used in vehicles like the Prius and Camry Hybrid, employs a sophisticated regenerative braking system. When the driver applies the brakes or releases the accelerator, the electric motor switches to generator mode, converting the vehicle's kinetic energy into electrical energy. This electricity is then stored in the high-voltage battery for later use.
The system is designed to seamlessly blend regenerative and friction braking, ensuring smooth deceleration while maximizing energy recovery. In stop-and-go traffic, this technology can recapture a significant amount of energy that would otherwise be wasted as heat in conventional braking systems.
Hydraulic hybrid technology: UPS delivery fleet implementation
While not as common in passenger vehicles, hydraulic hybrid systems offer another approach to energy recovery, particularly in heavy-duty applications. UPS has implemented this technology in some of its delivery vehicles. In a hydraulic hybrid system, the vehicle's kinetic energy is used to pressurize hydraulic fluid, which is then stored in an accumulator. This stored energy can be released to assist with acceleration, reducing the load on the engine.
Hydraulic hybrid systems can capture and release energy more quickly than electric hybrids, making them well-suited for vehicles that frequently start and stop, such as delivery trucks or garbage collection vehicles.
Flywheel energy storage systems: Porsche 919 hybrid racing application
In the world of high-performance racing, flywheel energy storage systems have gained traction as an alternative to battery-based hybrids. The Porsche 919 Hybrid, a Le Mans prototype race car, utilized a flywheel system to store and release energy. When the car brakes, the kinetic energy is used to spin up a carbon-fiber flywheel to extremely high speeds. This stored rotational energy can then be converted back into electrical energy to power an electric motor during acceleration.
While flywheel systems are currently limited to racing applications, their ability to rapidly store and release large amounts of energy could potentially be adapted for use in future consumer vehicles.
Intelligent driving modes for optimal fuel efficiency
Modern hybrid vehicles often come equipped with various driving modes that allow drivers to tailor the vehicle's performance to their needs and preferences. These modes adjust factors such as throttle response, transmission behavior, and the balance between electric and gasoline power usage. By understanding and utilizing these modes effectively, drivers can significantly enhance their fuel efficiency.
EV mode: maximizing electric-only range in Hyundai Ioniq
The Hyundai Ioniq Plug-in Hybrid offers an EV mode that allows the vehicle to operate solely on electric power. When engaged, this mode prioritizes the use of the electric motor and battery, avoiding the use of the gasoline engine as much as possible. In the Ioniq, EV mode can provide up to 29 miles of electric-only range, making it ideal for short trips or urban commutes.
To maximize the benefits of EV mode, drivers should:
- Fully charge the battery before each trip
- Accelerate gently to avoid triggering the gasoline engine
- Plan routes that fall within the electric range
- Use regenerative braking to extend range
Eco mode: throttle and climate control optimization in Kia Niro
Eco mode, available in many hybrid vehicles including the Kia Niro, focuses on maximizing fuel efficiency across all driving conditions. When activated, this mode typically adjusts several vehicle systems:
- Throttle response is dampened to encourage smoother acceleration
- The transmission shifts at lower RPMs to keep the engine in its most efficient range
- Climate control system operation is optimized to reduce energy consumption
- Regenerative braking may be intensified to capture more energy
By making these adjustments, Eco mode can significantly improve fuel economy, especially in city driving conditions. Drivers should consider using this mode for their daily commutes or when fuel efficiency is a priority over performance.
Power mode: balancing performance and efficiency in Lexus RX Hybrid
While hybrid vehicles are primarily designed for efficiency, many models offer a Power or Sport mode for situations where additional performance is desired. The Lexus RX Hybrid, for example, features a Power mode that sharpens throttle response and allows the electric motor to provide maximum assistance to the gasoline engine.
In Power mode, the vehicle prioritizes performance over efficiency, which can result in higher fuel consumption. However, this mode can be useful in situations such as merging onto highways or climbing steep hills. Drivers should use Power mode judiciously, reserving it for situations where the extra performance is truly needed.
Battery management and thermal regulation in hybrid vehicles
The high-voltage battery pack is a critical component of any hybrid vehicle, and its proper management is essential for maintaining optimal performance and longevity. Modern hybrid systems employ sophisticated battery management systems (BMS) that monitor and control various aspects of battery operation, including charge level, temperature, and overall health.
Thermal regulation is particularly crucial for hybrid batteries. Extreme temperatures, both hot and cold, can significantly impact battery performance and lifespan. To address this, hybrid vehicles use various cooling and heating systems to maintain the battery pack within an optimal temperature range. For example, some vehicles use liquid cooling systems that circulate coolant through the battery pack, while others employ air cooling or a combination of both.
The BMS also manages the charging and discharging cycles of the battery to prevent overcharging or deep discharging, both of which can reduce battery life. In plug-in hybrid vehicles, the system may also control charging rates and times when connected to external power sources, allowing for features such as scheduled charging during off-peak electricity hours.
Proper battery management is key to ensuring the long-term reliability and efficiency of hybrid vehicles. Advanced thermal regulation and charge management systems can extend battery life well beyond 100,000 miles in many cases.
Future trends: mild hybrids and 48V systems in fuel efficiency
As automakers continue to seek ways to improve fuel efficiency across their vehicle lineups, mild hybrid systems and 48-volt electrical architectures are gaining traction. These technologies offer many of the benefits of full hybrid systems but at a lower cost and with less complexity, making them attractive options for a wide range of vehicle types.
Mild hybrid systems, such as those used in some Audi and Mercedes-Benz models, typically employ a small electric motor-generator that can provide a power boost during acceleration and recover energy during braking. While these systems cannot power the vehicle on electricity alone, they can significantly improve fuel economy, especially in city driving conditions.
The shift to 48-volt electrical systems allows for the implementation of more powerful electric accessories and mild hybrid components without the need for the high-voltage systems used in full hybrids. This higher voltage enables the use of more efficient electric pumps, compressors, and other components that were traditionally driven by the engine, further reducing fuel consumption.
As these technologies mature and become more cost-effective, they are likely to be adopted across a broader range of vehicles, from compact cars to full-size SUVs. This widespread implementation of mild hybrid technology could lead to significant improvements in overall fleet fuel efficiency in the coming years.
The integration of mild hybrid systems and 48-volt architectures represents a pragmatic approach to improving fuel efficiency across diverse vehicle segments, potentially bridging the gap between conventional vehicles and full hybrids or electric vehicles.
By embracing these advanced technologies and understanding how to leverage them effectively, drivers can significantly reduce their fuel consumption and environmental impact without sacrificing the convenience and flexibility of traditional gasoline-powered vehicles. As hybrid technology continues to evolve, it promises to play a crucial role in the transition towards more sustainable transportation options.