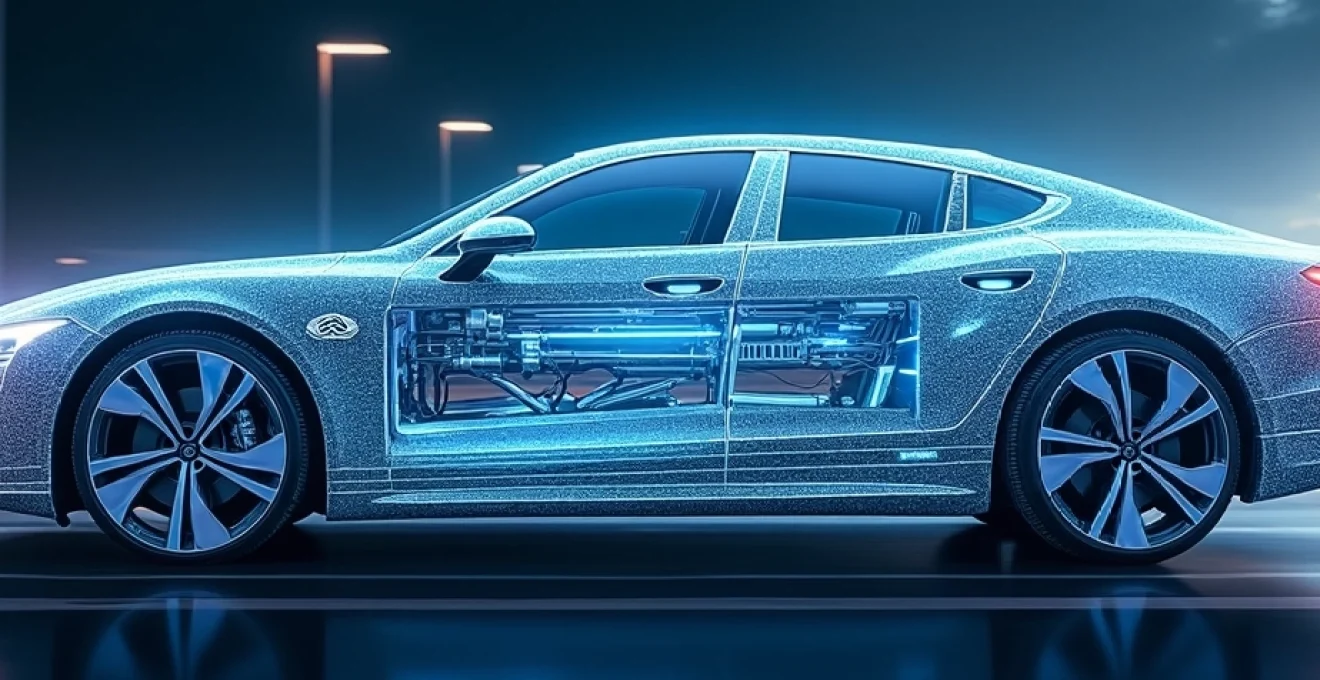
The automotive industry is undergoing a significant transformation, with hybrid vehicles at the forefront of this revolution. These innovative machines blend the efficiency of electric motors with the reliability and range of traditional combustion engines, offering a compelling solution for environmentally conscious drivers. As we navigate the transition towards cleaner transportation, hybrid technology serves as a crucial stepping stone, providing improved fuel economy and reduced emissions without compromising on performance or convenience.
Evolution of hybrid powertrain technologies
The journey of hybrid vehicles began in the late 20th century, with pioneering models like the Toyota Prius paving the way for widespread adoption. Since then, hybrid powertrain technologies have evolved rapidly, becoming more sophisticated and efficient with each passing year. Today's hybrid systems seamlessly integrate electric motors with internal combustion engines, utilizing advanced control algorithms to optimize power delivery and fuel consumption.
One of the key advancements in hybrid technology has been the development of more powerful and compact electric motors. These motors now provide significant torque, allowing for improved acceleration and the ability to operate in electric-only mode for longer distances. Additionally, battery technology has made great strides, with lithium-ion batteries replacing older nickel-metal hydride units, offering higher energy density and faster charging capabilities.
The integration of regenerative braking systems has also played a crucial role in the evolution of hybrid powertrains. These systems capture kinetic energy during deceleration, converting it into electrical energy to recharge the battery. This not only improves overall efficiency but also reduces wear on traditional friction brakes, extending their lifespan.
Parallel vs. series hybrid architectures
Hybrid vehicles can be broadly categorized into two main architectures: parallel and series hybrids. Each configuration offers distinct advantages and is suited to different driving scenarios.
Parallel hybrids, the most common type, allow both the electric motor and the internal combustion engine to directly power the wheels. This configuration offers flexibility, as the vehicle can run on electric power alone, combustion engine power alone, or a combination of both. Parallel hybrids are particularly efficient for highway driving, where the combustion engine can operate at its optimal speed.
Series hybrids, on the other hand, use the electric motor as the sole means of propulsion. The combustion engine serves only as a generator, producing electricity to charge the battery or power the electric motor. This setup is more efficient in stop-and-go traffic and urban environments, where frequent acceleration and deceleration occur.
Toyota Hybrid Synergy Drive system
Toyota's Hybrid Synergy Drive (HSD) system is a prime example of a sophisticated parallel hybrid architecture. It utilizes a power-split device that allows the system to operate as a parallel hybrid, series hybrid, or pure electric vehicle, depending on driving conditions. The HSD system continuously monitors vehicle speed, throttle position, and battery charge to determine the most efficient power source.
The HSD system's ability to seamlessly transition between power sources contributes to its exceptional fuel efficiency. In city driving, where speeds are lower and stops are frequent, the electric motor takes precedence. During highway cruising, the gasoline engine becomes the primary power source, with the electric motor providing additional power when needed, such as during overtaking maneuvers.
Honda's Integrated Motor Assist (IMA)
Honda's Integrated Motor Assist (IMA) system represents a simpler parallel hybrid approach. In this configuration, a thin electric motor is sandwiched between the engine and transmission. The IMA system primarily relies on the gasoline engine for propulsion, with the electric motor providing additional power during acceleration and allowing for engine shut-off during stops.
While less complex than Toyota's HSD, Honda's IMA system offers improved fuel efficiency and reduced emissions compared to conventional vehicles. It's particularly well-suited for smaller vehicles where packaging space is at a premium, as the compact design allows for easier integration into existing vehicle platforms.
Volvo's T8 Twin Engine configuration
Volvo's T8 Twin Engine system represents a more powerful hybrid configuration, often referred to as a through-the-road hybrid. This setup combines a turbocharged and supercharged gasoline engine driving the front wheels with an electric motor powering the rear wheels. The result is a vehicle with all-wheel drive capability and impressive performance characteristics.
The T8 system allows for pure electric driving for short distances, making it ideal for urban commutes. When maximum power is required, both the gasoline engine and electric motor work in tandem, delivering exceptional acceleration. This configuration demonstrates how hybrid technology can be applied not just for efficiency, but also to enhance vehicle performance and driving dynamics.
Gm's Voltec technology in extended-range EVs
General Motors' Voltec technology, featured in vehicles like the Chevrolet Volt, blurs the line between hybrid and electric vehicles. This system operates primarily as an electric vehicle for a certain range (typically around 50 miles) before switching to a series hybrid mode where a gasoline engine generates electricity to extend the driving range.
The Voltec system addresses range anxiety associated with pure electric vehicles while still allowing for significant electric-only driving. This configuration is particularly beneficial for drivers who have short daily commutes but occasionally need to travel longer distances without stopping to recharge.
Regenerative braking and energy recovery systems
Regenerative braking is a cornerstone technology in hybrid vehicles, significantly contributing to their efficiency. When a driver applies the brakes or decelerates, the electric motor acts as a generator, converting kinetic energy into electrical energy that is stored in the battery. This process not only recaptures energy that would otherwise be lost as heat in conventional braking systems but also reduces wear on the mechanical brakes.
Advanced regenerative braking systems can recover up to 70% of the vehicle's kinetic energy, dramatically improving overall efficiency, especially in urban driving conditions with frequent stops. Some hybrid vehicles allow drivers to adjust the level of regenerative braking, enabling a one-pedal driving experience in high regeneration modes.
Beyond regenerative braking, hybrid vehicles employ various other energy recovery systems. For example, some models capture waste heat from the exhaust system to warm the engine and cabin more quickly, reducing the load on the engine and improving fuel efficiency. Others use thermoelectric generators to convert heat from the exhaust into electricity, further enhancing energy recovery.
Plug-in Hybrid Electric Vehicles (PHEVs) and grid integration
Plug-in Hybrid Electric Vehicles (PHEVs) represent the next step in hybrid technology, offering the ability to charge the battery from an external power source. This feature allows PHEVs to operate as pure electric vehicles for longer distances, typically ranging from 20 to 50 miles, before switching to hybrid mode. The ability to plug in and charge from the grid provides greater flexibility and can significantly reduce fuel consumption for drivers with access to charging infrastructure.
PHEVs play a crucial role in the transition to fully electric vehicles, offering a bridge technology that combines the benefits of electric driving with the range assurance of a gasoline engine. As charging infrastructure continues to expand, PHEVs become an increasingly attractive option for consumers looking to reduce their carbon footprint without sacrificing convenience.
BMW i3 REx: Range Extender concept
The BMW i3 with Range Extender (REx) offers an innovative approach to plug-in hybrid technology. Unlike traditional PHEVs, the i3 REx is primarily an electric vehicle with a small gasoline engine that serves solely as a generator to extend the driving range. This configuration allows for a larger battery and more powerful electric motor compared to conventional PHEVs, prioritizing electric driving while still providing the security of extended range when needed.
The i3 REx demonstrates how PHEVs can be designed to maximize electric driving while still addressing range anxiety. This approach is particularly suitable for urban dwellers who primarily rely on electric power but occasionally need to travel longer distances.
Mitsubishi Outlander PHEV's Vehicle-to-Grid (V2G) capability
The Mitsubishi Outlander PHEV showcases advanced grid integration features, including Vehicle-to-Grid (V2G) capability. This technology allows the vehicle to not only draw power from the grid but also feed power back, essentially turning the vehicle into a mobile energy storage unit. V2G technology has the potential to play a significant role in grid stabilization and renewable energy integration.
During peak demand periods, V2G-enabled vehicles can supply power to the grid, helping to balance load and potentially reducing the need for costly peaker plants. Conversely, these vehicles can charge during off-peak hours when electricity is cheaper and more likely to come from renewable sources. This bidirectional flow of energy represents a paradigm shift in how we think about vehicle-grid interactions.
Chevrolet Volt's battery management and charging strategies
The Chevrolet Volt, a pioneering PHEV, employs sophisticated battery management and charging strategies to optimize performance and longevity. The Volt's system allows drivers to select from multiple charging modes, including immediate charging, delayed charging to take advantage of off-peak electricity rates, and location-based charging preferences.
Additionally, the Volt's battery management system reserves a portion of the battery capacity to ensure consistent performance in hybrid mode, even when the battery appears "depleted" for all-electric driving. This approach balances the benefits of electric driving with the need for reliable hybrid operation, ensuring a seamless transition between modes.
Toyota Pprius prime's dual motor generator system
The Toyota Prius Prime, the PHEV version of the iconic Prius, features a dual motor generator system that enhances both efficiency and performance. This setup allows for improved electric-only operation, including higher speeds and quicker acceleration in EV mode compared to the standard Prius hybrid.
The Prius Prime's system also enables more efficient regenerative braking and smoother transitions between electric and hybrid modes. By optimizing the interplay between its two motor generators, the Prius Prime achieves impressive fuel economy in both electric and hybrid operation, setting a high bar for PHEV efficiency.
Hybrid powertrain control strategies and optimization
The heart of any hybrid vehicle lies in its control system, which orchestrates the complex interplay between the electric motor, internal combustion engine, and battery. Advanced control strategies utilize real-time data from numerous sensors to make split-second decisions on power distribution, ensuring optimal efficiency and performance under varying driving conditions.
Machine learning and artificial intelligence are increasingly being employed to refine these control strategies. By analyzing patterns in driving behavior, route characteristics, and energy consumption, these systems can predict optimal power management strategies, further improving efficiency. Some hybrid vehicles even use GPS data to anticipate terrain changes and adjust power delivery accordingly, such as reserving battery power for upcoming uphill sections.
Thermal management is another critical aspect of hybrid powertrain optimization. Sophisticated cooling systems ensure that batteries, electric motors, and power electronics operate within their optimal temperature ranges, maximizing efficiency and longevity. Some hybrids use the air conditioning system to cool the battery during charging, ensuring faster and more efficient charging cycles.
Future trends: mild hybrids and 48V systems
As the automotive industry continues to evolve, mild hybrid systems and 48V electrical architectures are gaining prominence. These technologies offer many of the benefits of full hybrid systems at a lower cost and with easier integration into existing vehicle platforms.
Mild hybrid systems typically cannot propel the vehicle on electric power alone but provide significant assistance to the internal combustion engine, enabling features like extended start-stop functionality, torque boost during acceleration, and more efficient regenerative braking. The adoption of 48V systems allows for more powerful electric motors and the electrification of power-hungry accessories, further improving overall vehicle efficiency.
Mercedes-Benz EQ Boost technology
Mercedes-Benz's EQ Boost technology exemplifies the potential of advanced mild hybrid systems. This integrated starter-generator (ISG) system, operating on a 48V electrical system, provides a power boost of up to 21 horsepower and 184 lb-ft of torque. Beyond performance enhancement, EQ Boost enables coasting with the engine off, near-instantaneous engine restarts, and highly efficient energy recuperation.
The EQ Boost system's compact design allows for easy integration into various vehicle models, from compact cars to luxury sedans. This flexibility demonstrates how mild hybrid technology can be widely applied across a manufacturer's lineup, contributing to significant fleet-wide efficiency improvements.
Audi's MHEV (mild hybrid electric vehicle) platform
Audi's MHEV platform, available in both 12V and 48V configurations, showcases the scalability of mild hybrid technology. The 48V system, in particular, offers substantial benefits, including a coasting function that allows the engine to shut off completely at speeds between 34 and 99 mph, potentially saving up to 0.7 liters of fuel per 100 kilometers.
Audi's implementation also includes an electric compressor that provides near-instantaneous torque, eliminating turbo lag and improving responsiveness. This demonstrates how mild hybrid technology can enhance both efficiency and performance, making it an attractive option for a wide range of vehicle types.
Mazda M Hybrid electrification system
Mazda's M Hybrid system takes a unique approach to mild hybridization, focusing on seamless integration and natural driving feel. The system uses a belt-driven integrated starter generator (ISG) coupled with a 24V lithium-ion battery. While less powerful than some 48V systems, Mazda's approach prioritizes efficiency gains in real-world driving conditions.
The M Hybrid system extends the engine-off period during deceleration and stop-start operations, contributing to improved fuel economy, especially in urban driving. Mazda's focus on the driving experience ensures that the hybrid system's operation is nearly imperceptible to the driver, maintaining the brand's reputation for engaging dynamics while improving efficiency.
As hybrid technology continues to evolve, we can expect to see further innovations in powertrain design, energy management, and grid integration. The lines between mild hybrids, full hybrids, plug-in hybrids, and fully electric vehicles will likely continue to blur, offering consumers an increasingly diverse range of efficient and environmentally friendly transportation options. The ongoing development of hybrid technologies plays a crucial role in the automotive industry's journey towards a more sustainable future, bridging the gap between traditional combustion engines and the electric revolution.